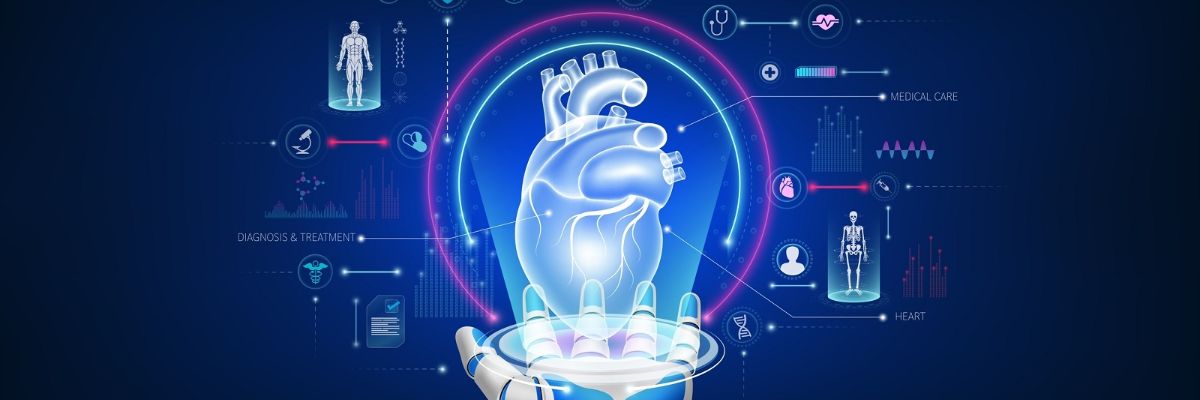
Heart replacements, custom-made. What can tissue engineering offer?
15. 05. 2023
Over the course of a human life, blood vessels, skin, bones, and joints accumulate wear and tear. Tissue engineer Lucie Bačáková is involved in coming up with the most elegant way to replace the damaged body parts. Her team’s research has been supported by the prestigious Academic Award of the CAS, which will provide funding for the next six years.
A regular day at the cardiologist’s. “Looks like you’ll be needing a new heart,” the doctor informs their patient before opening a database of available artificial organs on their computer. “I’ll prescribe you a size M. That should fit you. I’ll order it right away, and once it’s delivered to our hospital, you can come in for the replacement,” they add, the patient nodding along to it all, unperturbed. After all, today, every third person has some kind of (bio)artificial body part.
Could this be the melody of a not-too-distant future? According to Lucie Bačáková from the Institute of Physiology of the CAS, a similar database of artificially developed organs could easily exist in approximately fifty years.
“But I actually hope that patients will not – with rare exceptions – need them, because doctors will be able to regenerate damaged tissues effectively thanks to advancements in medical care,” the researcher notes.
Test-tube brains
But that is of course no reason not to work on developing artificial hearts, lungs, kidneys, and other vital body organs. On the contrary! In fact, they could be a major contribution to advancing the treatment of various diseases. All that would be needed to develop them would be for them “to float” in test tubes and Petri dishes instead of inside the human body.
“While we used to think of the creation of artificial organs for patients as the benchmark of tissue engineering, today the main trend is the attempt to prepare perfect models of organs and tissues cultured in vitro, i.e., for research purposes for the development and improvement of new pharmaceuticals. Scientists could thus finally stop using animals for testing,” Bačáková explains. As a rabbit lover herself, she would very much welcome this change.
In the lab, researchers would have everything at their disposal: engineered skin for the testing of cosmetics, blood vessels and hearts to artificial brains for the testing of drugs for neurological diseases.
“But these models would have to be pretty darn perfect – even better than organs designed to be implanted in human bodies. The human body works like a bioreactor – even an imperfect construct ends up being ‘baked’ or finished, to a certain extent. However, in a test tube, nothing like that happens,” the researcher points out.
The search for the Holy Grail
The year is 1953. A group of scientists and surgeons are celebrating the first successful insertion of an artificial blood vessel in a patient. A polymer tube had been inserted into his body. And from then on, tubes are pretty much what researchers stuck to.
Though seventy years have passed since that operation, there is still a lack of functional small-diameter blood vessel replacements on the market. These are the ones that are the most needed, given the high incidence of cardiovascular diseases.
“Developing blood vessels with a diameter of less than six millimetres is considered the holy grail of tissue engineering,” Bačáková says. She herself has been searching for it for the past twenty years. And in that time, she and her colleagues have done many trial-and-error runs.
Synthetic polymers, which have proven successful in replacing large blood vessels, have failed where small ones are concerned – the passive rubber tubes, as the researcher jokingly calls the replacements, get narrower over time in the patient’s body and thus the patient is in danger of thrombosis.
But then the researchers got an idea – what if they borrowed tiny blood vessels from a pig or perhaps a sheep? So they got to work. First, they had to decellularise the animal tissue, i.e., remove the cells, so that the human body wouldn’t reject the tissue. This left them with only the intercellular matter, arranged into the shape of a tube, which they then attempted to inlay with individual layers of the blood vessel wall from the patient’s stem cells.
This process is already looking promising, but it is not the Holy Grail, as the results are still less than ideal. For instance, there is still a risk of an immune reaction on the part of the patient and the possible transmission of pathogens.
“In addition to the polymer substitutes and animal-derived blood vessels, we have of course also tested the possibility of using the patient’s own blood vessels. However, for the patient this meant undergoing an extra operation, and even technically, this solution did not meet our expectations,” the researcher explains.
In fact, one of the few blood vessels which a person can “spare” a part of, i.e., the vena saphena (the superficial vein of the lower limb), is subjected to excessive mechanical strain in the position of the coronary arteries which nourish the heart and most often need replacing.
“Under the tremendous pressure which is found near the heart, it inflates like a balloon. We tried to prevent this by using an external stent – with some success, but the technical aspect was complicated,” Bačáková notes. However, even after these experiences, she has definitely not given up her search for the grail in the form of a small-diameter vascular replacement. It occurred to her that perhaps the route to it could be by way of subcutaneous fat or the umbilical cord. Or, to be specific, by way of the so-called mesenchymal stem cells from these tissues.
Samples of Ti6AI4V titanium alloy, which is used for the construction of joint replacements, with different surface treatments. (CC)
Fat and Wharton’s jelly
“There is a number of people who undergo liposuction voluntarily for cosmetic reasons. In comparison to saphenous vein or bone marrow extraction, liposuction is a relatively uncomplicated procedure. You just stick a needle in, suck out some of the fat, and voilà,” the researcher explains with a bit of hyperbole. In fact, subcutaneous fat, found just beneath the skin, is a great source of stem cells for researchers. “From these stem cells, we can then build the physiological layers of the vascular wall into a tube made of synthetic polymers already approved for clinical use. And hopefully, it will result in a narrow blood vessel,” Bačáková says of her plans.
The tried-and-tested polymer would serve as a scaffold that the researchers would additionally reinforce with cells. In fact, the mechanical properties of such a stent should be significantly better than those of non-cell-seeded polymer tubes. Bačáková would also like to use a similar process to create a heart valve replacement. In that particular case, using a precisely defined synthetic material and embedding it with cellular components seems to be the most promising option.
“I am working with what is already available. The prehistoric man also looked around, spotted some flint, a stone, and an oak stick, and constructed a spear out of it. In my case, I go about it the same way – I try to combine tried-and-tested materials and methods to create something new,” explains the avid reader of novels by Eduard Štorch, known for stories set in prehistoric Bohemia during the Stone and Bronze Age.
And stem cells are intrinsic to her research. In addition to the readily available subcutaneous fat, another rich source of stem cells is Wharton’s jelly, a gelatinous substance within the umbilical cord. However, this useful tissue usually ends up in biological waste, which is a great pity, according to Bačáková. She would thus like to isolate stem cells from the umbilical cords of just born babies of anonymous donors, which she would then use to try to “revive” the synthetic alternatives.
“In this case, however, we wouldn’t be working with the patient’s own tissue. According to previous research, umbilical cord stem cells are not very immunogenic. In other words, the host organism shouldn’t reject them,” Bačáková explains. Researchers have yet to test this hypothesis properly though. And should it not be successful, there is still liquid nitrogen to try out.
“It is possible to preserve cells in liquid nitrogen for many decades. Hypothetically then, if doctors stored the umbilical cords of every baby born in nitrogen, or just the blood from that tissue, then every individual would be able to use their own stem cells to help them treat various diseases in their old age,” the researcher speculates, adding that science has been implementing this technology for some time now – but not yet routinely.
PRAEMIUM ACADEMIAE |
Dreams of artificial cartilage
Of course, narrow blood vessels are not the only hot topic for tissue engineers. Cartilage reconstruction carries a similar hallmark of unattainability in their eyes. For now, joints are replaced by doctors without the cartilage, leaving in its place only an artificial, cell-free joint surface.
“My dream is to create, on the basis of artificial materials and stem cells, the entire osteochondral interface, which is the part where bone tissue transitions into cartilage,” Bačáková says.
If successful, the patient would thus not only receive a titanium knee, but a functional replacement that would simulate both bone and cartilage tissue. Embedding the cellular component in a composite of synthetic or natural polymer, ceramic, and metal is the way to make this dream become a reality, according to the researcher.
Besides, she has extensive experience in improving joints and their ligaments. For many years, she and her team worked on surface treatments for metals to make them more suitable to the bone ends adjacent to the joints being replaced. They tried to make sure that the metal surface had optimal roughness, adequate wetting, and electrical charge along with a sufficient amount of pores and nooks and crannies for the bone cells to crawl into. In short, they tried to accommodate the material as much as possible so that it would take hold well.
And indeed, the cells were sold. Lucie Bačáková and her colleagues have thus been successful in developing various innovations that have increased the lifetime of artificial thumb and hip joints, with several patents to their credit.
But these individual successes have certainly not left the proactive researcher resting on her laurels. In addition to her work on artificial blood vessels, heart valves, and cartilage, she is also searching for new materials for joint replacements and fillers for bone defects that would be more suitable for bone tissue. However, as she is a trained physician and her team is made up entirely of biologists, she cannot do without collaborating with top materials engineers, such as physicists, chemists, and mechanical engineers.
“Tissue engineering is a textbook example of an interdisciplinary field. Unfortunately, though, the experts from all the required fields cannot fit under one roof. So we often travel with the polymer we are researching to colleagues around Prague, sometimes to Ostrava, or even to Brussels. Or they come to us with an idea they have,” the researcher notes.
Cultivation container with cell culture for use in tissue engineering. (CC)
You can’t sweep away soot from inside your body
Years ago, for instance, experts from the Czech Technical University came to Bačáková at the Institute of Physiology of the CAS in Krč with the idea to test the possibility of using carbon composites to replace bone tissue. Since their mechanical and chemical resistance had been proven in the construction of aircraft hulls and space rockets, why not try the composites for human joints?
“We discovered that carbon composites are lighter and more similar in properties to bone in comparison to the widely used titanium. It looked very promising. But despite all the coating and strengthening, particles were falling out of them. In a machine, it doesn’t matter – you sweep the soot away from time to time and it keeps working. But in the body, this is unfeasible,” Bačáková explains.
That’s why researchers moved away from carbon composites. The patients evaded the “soot” in the body, but not poisonous substances. Not even titanium, which still plays a leading role in joint replacements today, is completely innocent. What’s more, chemists have to add a small percentage of aluminium and vanadium to the prostheses of the most stressed joints to tune their mechanical properties. And this pair of substances is known to be quite toxic.
“When it comes to knee replacements, people today don’t expect only the ability to walk somewhat decently. No, they want to be able to go skiing in the Alps. So they have enormous demands on the new joint,” the researcher notes, explaining why the titanium required improvement.
But how can a person live with poisonous metals in their body? For many years, they have no effect on the organism, but... There is no more corrosive and aggressive environment than the biological one. The cells keep attacking the implant, they eat away at it and want to rebuild it, attacking it with all kinds of enzymes. The vicinity of the new joint is constantly “on fire”. When physical exertion is added, for example alpine skiing, the crystalline lattice of the alloy breaks down in a number of years and aluminium and vanadium are then released into the body.
And since both of these metals are toxic to many important organs, especially the nervous system, the patient can be at risk of certain neurodegenerative diseases such as Alzheimer’s. So for researchers, the search for safer improvements of titanium has long been the order of the day. And the result? So far, the most promising has proven to be an alloy of titanium, niobium, and tantalum, which chemists have tentatively coined using the acronym TNT.
Despite its name, this variant should be significantly more stable. However, as has already been mentioned, even titanium itself is not entirely untainted. Unfortunately, replacements for extremely stressed joints such as knees or hips simply cannot do without it.
3D-printed organs
Besides bones, blood vessels, and heart valves, Bačáková is also interested in wound healing: those that do not heal and those that conversely regenerate too much, because this can also be detrimental, resulting in raised scars.
“My team and I are trying to find out what is biochemically missing or present in these sections of the skin, i.e., what the exact cause of the problem is,” the researcher explains. Tissue remnants from surgery are being used for research. However, their size is usually not large enough and they are often contaminated with microbes that are normally present on the skin.
But if researchers are able to successfully isolate and multiply the original cells from scars and wounds, they will have essentially won. A 3D printer can then be put to use to “repair” the problematic section found on the skin.
“We want to develop a method of 3D bioprinting of collagen or synthetic matrices with cells, the advantage of which is mainly higher productivity,” Bačáková says. Some of her colleagues even see so-called bioprinting as the future of tissue engineering and plan to print entire tissues complete with vasculature, bones, and even organs.
“Whether it proves successful or not, I still advise everyone to go easy on their bodies and take care of them. Evolution has had three-and-a-half billion years to create all the human body parts, while we have only a mere few grant years to invent replacements. Not even the best tissue engineer can compete with nature,” says the winner of the Academic Award of the CAS, adding, “Natural tissue will simply always be more sophisticated!”
doc. MUDr. LUCIE BAČÁKOVÁ, CSc. INSTITUTE OF PHYSIOLOGY OF THE CAS
She graduated from the First Faculty of Medicine of Charles University in Prague. Since 1984, she has been working at the Institute of Physiology of the CAS, where she initially focused on cardiovascular physiology. She heads the Laboratory of Biomaterials and Tissue Engineering there and is a leading Czech expert in these fields. She has published more than 200 articles in renowned scientific journals. |
The text was taken from the Czech issue of the A / Magazine of the CAS:
1/2023 (version for browsing)
1/2023 (version for download)
Prepared by: Radka Římanová, Division of External Relations, CAO of the CAS
Translated by: Tereza Novická, Division of External Relations, CAO of the CAS
Photo: Jana Plavec, Division of External Relations, CAO of the CAS, Shutterstock
The text and photographs marked (CC) are released for use under the Creative Commons licence.
Read also
- How to repair the spinal cord: Kristýna Kárová explores nerve cell recovery
- Bohemian Paradise Apples – The Station of Apple Breeding for Disease Resistance
- Plants contain an incredible wealth of chemicals, Tomáš Pluskal says
- Two dozen young scientists received the Otto Wichterle Award
- Threads of history: what can archaeological textiles reveal?
- Over 2,000 silver coins from the 12th century discovered near Kutná Hora
- The nanoworld of molecules and future electronics: Two Dioscuri Centers open
- Celebrating bees: understanding the role of pollinators and why they are at risk
- What makes up cosmic rays? A new method by a Czech physicist provides insight
- New training will help confront gender-based violence in academia
The Czech Academy of Sciences (the CAS)
The mission of the CAS
The primary mission of the CAS is to conduct research in a broad spectrum of natural, technical and social sciences as well as humanities. This research aims to advance progress of scientific knowledge at the international level, considering, however, the specific needs of the Czech society and the national culture.
President of the CAS
Prof. Eva Zažímalová has started her second term of office in May 2021. She is a respected scientist, and a Professor of Plant Anatomy and Physiology.
She is also a part of GCSA of the EU.