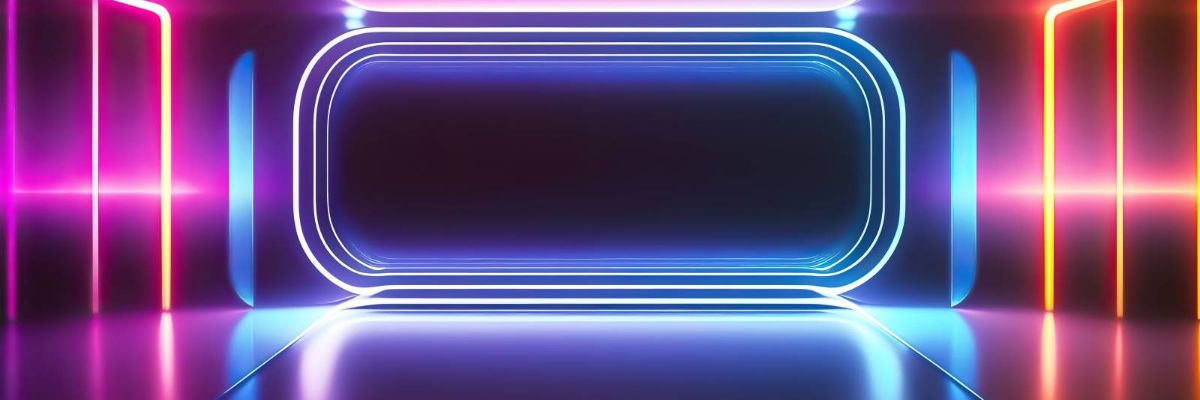
Light – Illuminating the invisible
15. 10. 2024
Candles, lamps, microscopes… These all help shed light on the world around us. Properly “tamed” light, however, can uncover previously unimaginable depths. Read our feature, first published in the 3/2024 (Czech) issue of A / Magazine, on how microscopes, endoscopes, and lasers are used at the service of science, below.
Sometimes, the lives of ordinary people change history, but sometimes, it is their deaths, too. On 25 November 1901, 51-year-old Auguste Deter was brought in for an examination to the Institution for the Mentally Ill in Frankfurt. She was confused, forgetful, experienced severe mood swings, and didn’t recognize her surroundings. She couldn’t remember where she lived or that she was married. The doctor in charge of her case was Alois Alzheimer.
Alzheimer was familiar with similar symptoms in much older patients, so Deter’s case captured his attention. He recorded her fragmented and confused responses in their conversations, carefully documenting the manifestations of her disease. When she died, he was given the unique opportunity to study her brain. Examining samples of it under a microscope, the doctor noticed significant changes – severe neuron loss and “tangles” formed by neurofibrils (filaments).
More than a century has passed since Alois Alzheimer’s time, and the field of microscopy has seen astonishing advancement. In addition to optical microscopes, the range now includes X-ray, electron, and atomic force microscopes, with scientists continuing to develop new types.
Although microscopy today can reveal previously unthinkable details, it comes with the disadvantage of having to treat samples – such as freezing or placing them in a vacuum, essentially “killing” them. This can cause issues when trying to study living matter – for instance, when observing in real time what is happening deep in a diseased brain or how DNA molecules behave.
Two unique discoveries made by Czech scientists aim to bridge these limitations of modern microscopy. The first is holographic endoscopy designed for deep-brain in vivo observations of nerve cell activity. The second is the nanofluidic scattering microscopy method, which uses light to observe individual biomolecules in their native state.
Tomáš Čižmár’s team in the Complex Photonics Lab. From left: Petra Kolbábková, Miroslav Stibůrek, Tomáš Čižmár, and Hana Uhlířová. Other group members include Tomáš Tyc, Tomáš Pikálek, and Bára Krbková.
From optical tweezers to the brain
Imaging the brain remains a significant challenge even today. Creating a probe that can penetrate deep enough to visualize the synapses between individual neurons is extremely difficult. Current imaging methods risk damaging delicate brain tissue.
A special holographic endoscope, utilizing the unique properties of light transmitted via hair-thin optical fibers, could offer a potential breakthrough. The development is led by Tomáš Čižmár, who works at the Institute of Scientific Instruments of the Czech Academy of Sciences (CAS) and also heads the Fiber Research and Technology Department at the Leibniz Institute of Photonic Technology.
“I actually stumbled upon brain research by accident. I’d never set out to study it specifically,” Čižmár recalls. He also came across the entire field of optics and photonics by chance, as he initially studied plasma physics at Masaryk University in Brno. However, a fateful excursion to the Institute of Scientific Instruments, specifically the Microphotonics Research Department led by Pavel Zemánek, changed his course.
“They were researching and developing optical trapping (optical tweezers). When I saw what light could do, my jaw dropped. I knew then and there this was what I wanted to do,” Čižmár says, joining Zemánek’s team as a Ph.D. student soon after.
Optical trapping is a technique that uses light to capture and manipulate microscopic objects, often micrometers in size. Optical tweezers are one of the most widely used types of optical trapping. Zemánek’s microphotonics research team has succeeded in commercializing some of these devices in cooperation with the company Meopta.
Čižmár also focused on optical trapping during his postdoc at the University of St. Andrews in Scotland. His task there was to create spatially structured optical fields that could be used for manipulating microobjects.
At the time, he was frustrated by how often he encountered aberrations when working with optical beams. Optical aberration causes a point not to appear in focus, but as a blurry spot with uneven intensity distribution. People with an eye condition called astigmatism experience a similar issue, which is corrected with glasses that have cylindrical lenses.
Čižmár spent a long time experimenting with different solutions, often in increasingly aberrated optical systems. “Eventually I realized that the solution is applicable to media that is completely optically random, such as optical fibers,” he recalls.
Prof. Mgr. TOMÁŠ ČIŽMÁR, Ph.D. Tomáš Čižmár studied physics at Masaryk University in Brno, focusing on optics in his doctoral studies. Even as a Ph.D. student, he worked at the Institute of Scientific Instruments of the CAS in Pavel Zemánek’s team, focusing on the development of optical tweezers. He completed his postdoc research in Scotland at the University of St. Andrews. From 2013 to 2017, Čižmár taught at the University of Dundee in Scotland, and since 2017, he has led the Fiber Research and Technology Department at the Leibniz Institute of Photonic Technology. He also teaches at the Friedrich Schiller University and heads the Complex Photonics Lab at the Institute of Scientific Instruments of the CAS. |
The taming of the light
Optical fibers are commonly used for high-speed internet transmission. They are immune to electromagnetic interference and transmit signals over longer distances with higher speed. Increasingly replacing the once-dominant metal wires, optical fibers are made from glass or, in some cases, plastic.
However, the focus of Čižmár’s research at St. Andrews wasn’t on speeding up the internet; it was on effectively “taming” light to create beautiful, aberration-free optical fields. One of the potential applications of this technology is imaging biological tissues – particularly brain matter. “Neuroscience seemed like a natural fit, as it was clear this was exactly what it lacked – the ability to peer deeper into the brain and reveal the fascinating things happening within,” Čižmár explains.
Soon after, Čižmár received an important job offer from the University of Dundee (2013–2017), followed by an ERC Consolidator Grant for a project titled LIFEGATE, amounting to two million euros. Simultaneously, he was awarded a grant from the European Regional Development Fund totaling seven million euros, with both grants running from 2017 to 2022.
“That was a rather hectic period, because I relocated from Scotland to Germany with my family, while one of the projects was conducted in Brno at my original home institute. I was working with and coordinating two teams at the same time, which was no easy feat,” Čižmár recalls. During this time, he was fully focusing on developing the holographic endoscope – a device that utilizes optical fibers to direct light beams.
At the starting point of the system is a laser roughly the size of a shoebox. It sends light through an optical cable to a set of lenses and mirrors. Here, the light refracts and is redirected to a computer-controlled holographic modulator about the size of a matchbox, which imparts the properties to the light that are needed for the intended use.
The modified light is then sent into a special multimode optical fiber (approximately one tenth of a millimeter wide) which is ready to penetrate animal tissue – in this case, the brain. Specifically, it targets the brain of a model organism for human disease, often a lab mouse. Animal models enable neuroscientists to better understand brain function, and the findings can be applied in further medical and pharmaceutical research.
OPTICAL TWEEZERS AND ATOMIC CLOCKS Light serves as both a tool and a subject of research for many scientific teams at the Czech Academy of Sciences, the scope of which could fill a book. “In our Microphotonics Research Department, for instance, we use optical traps to capture, manipulate, cool, or characterize both non-living and living microorganisms with light,” says Pavel Zemánek from the Institute of Scientific Instruments of the CAS. “Our work closely aligns with that of Ondřej Číp’s Coherence Optics Research Department, which uses light for highly precise measurements, cooling ions in atomic clocks, distributing stable optical frequencies across European communication networks, and even welding and micromachining.” |
The universe of a mouse’s brain
Working with mouse models is subject to strict ethical controls aimed at eliminating unnecessary suffering of the animals. The procedure for inserting the fiber, i.e., craniotomy (drilling a small hole into the skull), is done under anesthesia. The mouse does not feel the fiber insertion into its brain, and it can be conducted while the animal is active. Compared to previous endoscopic techniques, a major advantage of this method is the miniature size of the probe, which is far less invasive. Researchers use fluorescent tags to visualize neurons and other parts of the brain they need to observe. In simplified terms, cells and their surroundings are “stained” (this can be done via genetic manipulation of the sample or viral transfection – what then fluoresces is the protein, produced by the cell in response).
As the fiber moves through the mouse’s brain, an entire universe of interconnected neurons appears on the screen. “We can see various details, such as the dendrites extending from the neuron. Dendrites have spines – structures where neurons exchange information through synapses. It’s a dynamic structure, so many scientists are interested in how the cells activate, how individual parts of neurons are formed, how they establish connections, and how active they are,” Čižmár explains.
The holographic endoscope developed by Čižmár and his team is now ready for use. DeepEn, a spin-off company founded by the Czech scientist and his colleagues in Germany, is managing the transition to practical applications. They are now presenting the device at neuroscience conferences and hosting workshops aimed at explaining how it works. Various research institutions, particularly those focusing on brain research, neuron activity, and neural degeneration, have already expressed interest.
Though the project may appear to be completed, it is far from over. Čižmár and his colleagues are continuing to refine the instrument. The next milestone is to adapt the equipment to allow the mouse models free movement. Currently, the endoscope works reliably when the mouse has a holder with the probe attached to its head and it only runs on a pad under the holder. However, the flexibility and plasticity of the fiber theoretically allow for monitoring the brain’s activity in the animal’s natural environment – something that isn’t yet possible. When the animal moves at will, the light in the fiber clashes, distorting the transmitted information.
DEEP INTO THE BRAIN The holographic endoscope, developed by Tomáš Čižmár’s team, enables deep penetration into the brain to visualize individual neurons and their communication. A laser sends light via an optical cable to a device consisting of lenses and mirrors (1; photo below). Here, the light is split and directed toward a computer-controlled holographic modulator that imprints specific properties onto the light for the given application. The modified light is then fed into a multimode optical fiber about a tenth of a millimeter wide (2), which penetrates biological tissue – in this case, the brain of a mouse (3), a model organism for human diseases. Photo 4 shows the holographic endoscope device that is used in the lab. The commercial version of the endoscope, intended for neuroscientific or pharmaceutical research, is more compact and practical. You can see examples of it on the website of the spin-off company DeepEn. |
And what about using the holographic endoscope on a human brain? It would certainly be fascinating to observe the brain of a patient, like Auguste Deter mentioned before, in real-time. Technically, it is possible – with several obstacles at play. For instance, using fluorescent labeling in the human body (which involves manipulating a living organism) is complicated. “The benefit for humans, however, is evident. It lies primarily in imaging and studying a given disease in animal models,” Čižmár concludes.
Tamed light?
Light possesses unique physical properties, and the more we learn about it, the better we get at utilizing it. We’ve learned to use light to manipulate (and even sort) microobjects, transmit data, change the structure of materials with laser-bound light, and we’re even capable of using light in machining and cutting. But we still haven’t tapped into all the possibilities light has to offer.
“There are still plenty of gaps to fill. We’ve only tamed light to a certain extent. We still face the challenge of how to shape light effectively in both space and time. Achieving that would result in remarkable applications, particularly in medicine,” Čižmár adds.
Many scientists are trying to harness light and utilize its properties, particularly for medical research. Barbora Špačková from the Institute of Physics of the CAS approaches light from a slightly different angle. Yet in the long run, her research too could help patients like Auguste Deter.
The raw power of light
“For most of us, light is a tool that allows us to see. What’s surprising, though, is that in the nanoscale world where my research takes place, light is an incredibly crude and imprecise tool,” Špačková explains. That’s because the wavelength of light is about one hundred times larger than the objects she focuses on in her work. “It’s fascinating that we can trick light and use it not only for observing but also measuring and weighing nanoscale objects,” she adds.
Her primary interest is in biomolecules – the fundamental building blocks of all life. These molecules are up to one hundred thousand times smaller than dust particles and invisible to conventional optical microscopy. They are also incredibly fast. In their natural aqueous environment, they can move hundreds of nanometers within a single millisecond.
Ing. BARBORA ŠPAČKOVÁ, Ph.D. Barbora Špačková studied physical engineering at the Czech Technical University in Prague. As a PhD student, she worked at the Institute of Photonics and Electronics of the CAS on the development of optical biosensors (2005–2017). From 2017 to 2021, she was a research fellow at Chalmers University of Technology in Sweden, working in Christoph Langhammer’s group. She co-founded the Swedish spin-off company Envue Technologies, which focuses on the commercialization of nanofluidic scattering microscopy. Currently, she leads the Dioscuri Center for Single-Molecule Optics, initiated by the Max Planck Society, which started its operations on 1 July 2024. |
Just how can such a super-fast and invisible entity be observed and recorded? “It’s extremely difficult, like looking for a black cat in a dark room. And often, you’re not even sure if it’s there,” Špačková notes. This issue is often circumvented by fluorescent microscopy, which attaches a fluorescent marker to the observed molecule that then acts as a beacon in the dark. However, the marker can significantly influence the behavior of the studied molecule. Observing biomolecules without markers is difficult – but not impossible. Špačková has managed to overcome this hurdle.
Originally, Špačková’s research focus lay elsewhere. Like Tomáš Čižmár, a pivotal shift in her career took place abroad – at Chalmers University of Technology in Sweden, where she went as a postdoctoral researcher in 2017. She wanted to develop biosensors suitable for detecting chemical and biological substances, such as air pollutants or biomarkers in blood – a topic she had explored at her previous workplace, the Institute of Photonics and Electronics of the CAS.
One day, her colleagues in the Swedish lab showed her an optical phenomenon interfering with their project they wanted to eliminate. They didn’t know why it worked the way it did or what it might be good for. Špačková found the issue intriguing and decided to investigate it further. She delved into the relevant theory, analyzed various possibilities, and explored how light should behave in certain situations – such as when encountering a biomolecule.
The theory suggested that in this case, light could be capable of detecting the biomolecule. This led to the idea of creating a unique microscope that could visualize biomolecules in their natural aqueous environment. “I received incredible support from my supervisor at the time. He too thought that if it worked, it would be groundbreaking. He asked me what I needed – a lab? Time? Equipment? The idea was so appealing that he provided all the resources I needed to realize it,” Špačková says.
Light trapped in a pipe
Several months of intensive work gave rise to the prototype microscope. “The moment I first saw a DNA molecule in our microscope is something I’ll never forget. It happened to coincide with the same day the media released the very first photo of a black hole,” the researcher recalls, referring to 10 April 2019.
The scientists named it the Nanofluidic Scattering Microscopy method. Nanofluidics is the study of fluid flow at the nanoscale, and it’s a field that Christoph Langhammer’s team, which Špačková was part of in Sweden, specializes in.
A nanofluidic device can be likened to a miniature pipeline through which fluid flows. The “pipe” is made of glass and is constructed using electron-beam lithography (instead of the stylus used in traditional lithography, the structure is drawn with an electron beam).
To visualize the observed biomolecule in the “pipe,” Špačková employed the principle of light interference. When light waves interact, they influence each other, amplifying their effects. “The intensity of the two interacting light waves doesn’t simply add up – one and one can equal two, but just as well zero or four, in this case,” Špačková explains.
In size, the nanofluidic microscope is comparable to Čižmár’s holographic endoscope. Both fit within a space roughly the size of a smaller bathroom. The device developed by Špačková and her colleagues also consists of a set of mirrors and lenses through which laser light passes.
The sample of the fluid under examination (e.g., cell secretion, blood plasma) is applied to a nanofluidic chip. The chip is a square plate containing a series of larger microscopic channels that guide a drop of fluid into smaller nanofluidic channels. This is where the interaction, scattering, and interference of light with the molecule occur.
The sample of the examined fluid – whether it’s cell secretion or blood plasma – is applied to the nanofluidic chip. This square plate, roughly one centimeter by one centimeter in size, contains a series of larger microscopic channels that guide a drop of fluid into smaller nanofluidic channels. Here, the interaction, scattering, and interference of light with the molecule occur. The molecule is then visualized on a computer screen as a moving dark “blot.”
To the untrained eye, this may appear as a mere smudge, but according to Špačková, biophysicists and biochemists are thrilled. Not only does observing the movement and interactions of molecules brings unique insight into the life of biomolecules, but this method also enables the detailed analysis, measurement, and weighing of individual molecules.
As a result, the first promising collaborations with labs of research institutions and pharmaceutical companies are already in the making. A spin-off company, Envue Technologies, was founded in Sweden to facilitate the transfer of this discovery into practical use. Špačková is also working on the next generation of the instrument, which will offer both better resolution and additional functionalities, such as the ability to manipulate individual molecules or measure their charge.
Špačková is continuing the development in the Czech Republic, where she returned with her family in 2022. She found a home at the Institute of Physics of the CAS where, since July 2024, she has been leading the Dioscuri Center for Single-Molecule Optics, initiated and supported by the Max Planck Society. The new center will employ six scientists, and so far, doctoral students from abroad have been the primary applicants. Thanks to generous funding, the hired researchers will have adequate pay, which is not always a given in Czech science. The center aims to attract young researchers with a foundation in physics who also have knowledge of biochemistry, biophysics, and experience with microscopy or nano/microfluidics.
Across the nanoverse
“We are embarking on a journey across the biological nanoverse, like nanoscale explorers discovering the fundamental building blocks of life,” Špačková notes. But the work won’t stop at merely marveling at the hidden corners of the nanoworld. In collaboration with molecular biologists, her team plans to focus on specific issues related to molecular transport within cells and processes linked to the development of diseases such as Alzheimer’s.
While current brain research won’t help Auguste Deter, who passed away more than a century ago, the number of people facing a similar fate is continually growing. In the Czech Republic alone, an estimated 150,000 people suffer from Alzheimer’s disease, and by 2050, this number is expected to nearly double.
Whether scientists will be able to reverse this grim prediction remains uncertain. However, it is clear that with discoveries like the nanofluidic scattering microscope and the holographic endoscope, researchers may “shed light” on Alzheimer’s disease in ways previously unimaginable.
Written and prepared by: Leona Matušková, External Relations Division, CAO of the CAS
Translated by: Tereza Novická, External Relations Division, CAO of the CAS
Photo: Shutterstock; Jana Plavec
The text and photos are released for use under the Creative Commons license.
Read also
- A trapped state: The pandemic impact on public attitudes, trust, and behavior
- Aerial archaeology: Tracing the footsteps of our ancestors from the sky
- Archaeologists uncover ancient finds along Prague Ring Road
- Our microbiome largely depends on what we eat, says microbiologist Michal Kraus
- The ABCs of writing: Why did its invention mark a turning point for humankind?
- We learn, remember, forget… What can memory actually do? And can we outsmart it?
- New Center for Electron Microscopy in Brno opens its doors to global science
- The hidden lives of waste: What can we learn from waste workers and pickers?
- A unique lab is hidden right beneath Prague’s Vítkov Hill
- Renewables are a strategic investment in European security, scientists say
The Czech Academy of Sciences (the CAS)
The mission of the CAS
The primary mission of the CAS is to conduct research in a broad spectrum of natural, technical and social sciences as well as humanities. This research aims to advance progress of scientific knowledge at the international level, considering, however, the specific needs of the Czech society and the national culture.
President of the CAS
Prof. Eva Zažímalová has started her second term of office in May 2021. She is a respected scientist, and a Professor of Plant Anatomy and Physiology.
She is also a part of GCSA of the EU.