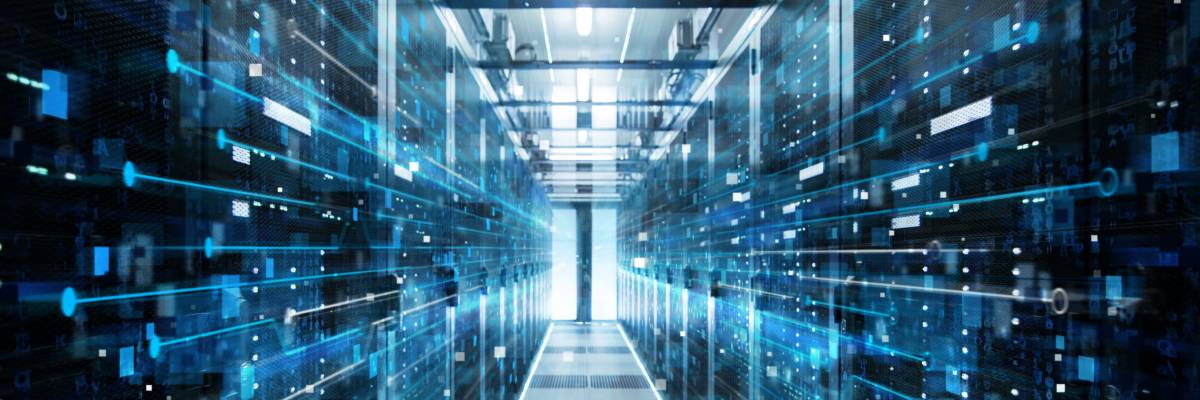
Altermagnetism has been experimentally confirmed
15. 02. 2024
In an article published in Nature, an international team of scientists breaks down the traditional idea of dividing magnetism into two branches – the ferromagnetic one, known for several millennia, and the antiferromagnetic one, discovered about a century ago. Researchers have now succeeded in directly experimentally demonstrating a third altermagnetic branch, theoretically predicted by researchers in Prague and Mainz several years ago.
We usually think of a magnet as a ferromagnet, which has a strong magnetic field that can keep a shopping list pinned to the fridge or that allows the electric motor in an electric car to function. The magnetic field of a ferromagnet is created when the magnetic field of millions of its atoms is aligned in the same direction. This magnetic field can also be used to modulate the electric current in IT components.
Limitations of the traditional magnetic branches for IT
At the same time, however, the ferromagnetic field poses principal limitations on the spatial and temporal scalability of these components. Thus, a significant research focus in recent years has been directed at the second, antiferromagnetic branch. Antiferromagnets are materials less known but much more common in nature, characterized by directions of the atomic magnetic fields on adjacent atoms alternating like white and black color on a chessboard. Thus, antiferromagnets as a whole do not create undesirable magnetic fields, but unfortunately, they are so anti-magnetic that they have not yet been actively used in IT.
Altermagnets combine “incompatible” merits
“The recently predicted altermagnets can combine the merits of ferromagnets and antiferromagnets, which were thought to be fundamentally incompatible, and also have other unique merits not found in the other branches,” says Tomas Jungwirth from the Institute of Physics of the Czech Academy of Sciences. Altermagnets can be thought of as magnetic arrangements where not only the directions of the magnetic fields on neighboring atoms alternate, but so does the spatial orientation of the atoms in the crystal (see the figure). However, the internal magnetic fields modulate the electric current in a similar way to ferromagnets. This combination of properties is potentially very attractive specifically for applications in future ultrascalable nanoelectronics.
In addition, altermagnetic candidates have been identified among more than 200 materials ranging from insulators and semiconductors to metals and superconductors. Research groups have investigated many of these materials in the past, but their altermagnetic nature has remained hidden from them.
The altermagnetic branch was theoretically predicted five years ago
Since 2019, the team from the Institute of Physics in Prague and the University of Mainz has published a series of articles theoretically identifying unconventional magnetic materials. In 2021, theorists predicted that these materials represent a third fundamental type of magnet, which they called altermagnets and whose crystal and magnetic structure is completely different from conventional ferromagnets and antiferromagnets (in the words of the physicists, altermagnets have completely different symmetries than ferromagnets and antiferromagnets).
Since altermagnetism opens broad and unprecedented research and application opportunities, the theoretical prediction was almost instantly followed by an outburst of follow-up studies by research groups from all around the globe. The next question then was when direct experimental evidence of altermagnetism would be available.
Experimental evidence was carried out on material considered for decades to be a “classical antiferromagnet”
The international team has now provided such evidence. The scientists decided to examine crystals of a simple two-element altermagnetic candidate – manganese telluride (MnTe). Traditionally, this material has been considered a classical antiferromagnet because the magnetic fields on neighboring manganese atoms point in opposite directions, and so they do not create an external magnetic field around the material.
But now, writing in Nature, the researchers have directly demonstrated altermagnetism in MnTe for the first time. They used theoretical predictions to navigate which direction to “shine the light” on high-quality MnTe crystals in a photoemission experiment.
The team measured band structures (maps that physicists use to describe the properties of electrons in crystals) on a synchrotron, using them to show that despite the absence of an external magnetic field, electronic states in MnTe are strongly spin-split. The scale and shape of the spin splitting exactly match the altermagnetic splitting predicted using quantum mechanical calculations. This is direct evidence that MnTe is neither a conventional antiferromagnet nor a conventional ferromagnet but belongs to a new altermagnetic branch of magnetic materials. The study made use of the expertise of researchers from the Institute of Physics of the Czech Academy of Sciences in collaboration with scientists from the Paul Scherrer Institute in Switzerland, University of Mainz in Germany, University of West Bohemia and Charles University in the Czech Republic, University of Linz in Austria, and University of Nottingham in Great Britain.
The discovery of altermagnetism has launched new directions in global research
“After the initial predictions and with the rapidly growing world-wide interest in altermagnetism, and given that many of the theoretically identified material candidates are well-known and broadly available, we knew that it was only a matter of time before the first direct experimental evidence would be discovered. We are glad that we could coordinate and be part of this initial work, which we have performed jointly with our colleagues from Czech, Swiss, Austrian, German, and British laboratories,” says Tomas Jungwirth from the Institute of Physics of the Czech Academy of Sciences, adding: “The discovery of altermagnetism has launched new directions in global research into new physical and material principles for highly scalable and energy-efficient IT components.” As can be seen, the discovery of altermagnetism in MnTe is only the beginning of exciting new prospects in magnetism.
More about the authors of the study:
Libor Šmejkal, a principle theory author on the Nature paper and a former PhD student in Prague and Mainz, is the winner of the Falling Walls Science Breakthroughs of the Year 2023 for his theory work on altermagnetism and non-dissipative nanoelectronics.
Dominik Kriegner, an experimental co-author from Prague, has received the Lumina Quaeruntur Fellowship of the Czech Academy of Sciences to develop the materials portfolio of altermagnetism.
Helena Reichlová, another Prague experimental physicist contributor to the Nature paper, has established a Max Planck Dioscuri Center for spin-caloritronic and magnonic research in altermagnets.
Tomas Jungwirth has started his second ERC Advanced Grant project focusing on applications of altermagnetism in spintronic IT. Altermagnetism is also among the central themes of the recently awarded 20MEUR project of the EU-funded Johannes Amos Comenius Programme, coordinated by Tomas Jungwirth.
In altermagnets, not only do the directions of spin polarization (shown in purple and blue) alternate on neighboring magnetic atoms, but so do the shapes of the atoms themselves (shown by the tilt of the electron densities in two different directions). The blue beam shows a photoemission experiment on a synchrotron that was used to demonstrate altermagnetism. Image credit: Libor Šmejkal, Anna Birk Hellenes.
Contact information:
Tomáš Jungwirth
jungw@fzu.cz
Libor Šmejkal
smejkall@fzu.cz
References:
Altermagnetic lifting of Kramers spin degeneracy
Krempaský*, L. Šmejkal*, S. W. D'Souza*, M. Hajlaoui, G. Springholz, K. Uhlířová, F. Alarab, P. C. Constantinou, V. Strokov, D. Usanov, W. R. Pudelko, R. González-Hernández, A. Birk Hellenes, Z. Jansa, H. Reichlová, Z. Šobáň, R. D. Gonzalez Betancourt, P. Wadley, J. Sinova, D. Kriegner, J. Minár, J. H. Dil, T. Jungwirth
Nature 626, 517–522 (2024)
DOI: 10.1038/s41586-023-06907-7
Read also
- How lakes connect to groundwater critical for resilience to climate change
- A unique method of rare-earth recycling can strengthen the material independence
- High-energy cosmic rays dominated by heavy METALS
- Genome Tool Developed at CAS Featured in PLoS Genetics
- Why do brain cancer cells steal mitochondria?
- Secrets of the Nano- World: a new comic book about nanotechnology
- Teen duo from Slovakia and Czechia named Global Winner for clean water solution
- Professor Pavel Hozák Receives the Paul Nakane Prize
- Neutrino is lighter than previously thought
- The Earth Prize 2025 goes to Czechia and Slovakia for pioneering water purifier
Contacts for Media
Markéta Růžičková
Public Relations Manager
+420 777 970 812
Eliška Zvolánková
+420 739 535 007
Martina Spěváčková
+420 733 697 112